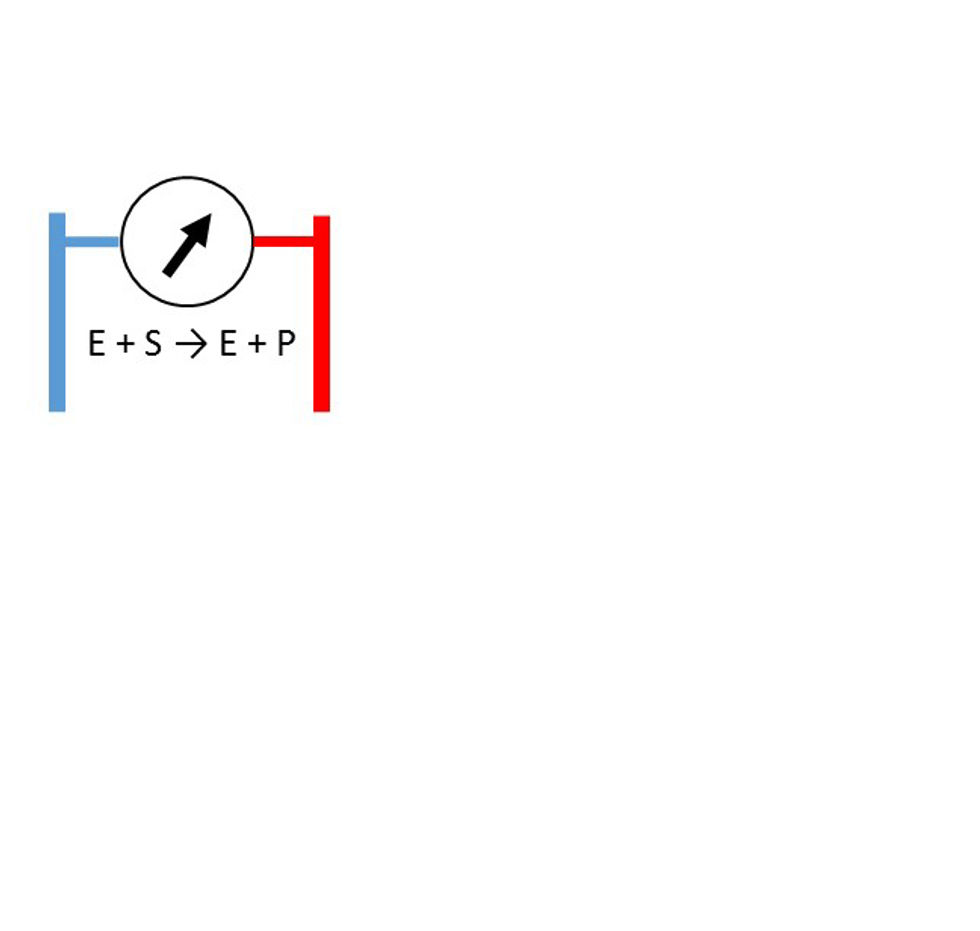
DROP DOWN MENU
Intro. Specific & Technical
A simple, inexpensive conductivity apparatus for
measuring enzyme-catalysed reactions
The electrical conductivity of liquids and solutions has been measured since about 1800, in the early days of electrical knowledge, so it is hardly a novel method! The first measurement with an enzyme was made in 1903.
Today it is very widely applied in chemistry, the water industry, environmental monitoring and the power generation industries. It has become routine and simple to assess the dissolved salt content of aqueous solutions (i.e. sea water, river water or boiler feed water) over a very wide range of concentrations. It does not tell you what the dissolved salts are.
Conductivity has, over the years, hardly been used in biochemical rate assays at all (see references at the end, where one early paper that sets the scene clearly is available online), for reasons given below, but has been widely used for monitoring ion content in chromatography. There has been recent activity with microdevices, including biochemical assays.
The electrical current is carried by positively & negatively charged ions in the solution. The type of ion, particularly it’s size and charge, has an effect on the conductivity of a solution.
Commercially available conductivity meters can be inexpensive (£40), but when they are, they are only capable of accurately measuring a limited range of conduction, usually where the conduction is low, i.e. reasonably pure water. ‘Research Grade’ conductivity meters are available that measure over a very wide range but these are expensive (at least 10 times more so than the simple ones).
Chemical reaction processes have been monitored using conductivity change for a long time. Thus the generalised reaction shown below can easily be followed:
A – B + H – O – H ---------> [A – O – H] - + B - H+
The + & - charges that are generated as a result of the reaction carry the current and the conductivity increases in direct proportion to the degree of reaction.
Biochemical reactions are fundamentally the same but need to be measured at much lower concentrations (100 – 1000-fold lower) that are relevant to concentrations of substrates found in living organisms. For this reason the apparatus needs to be much more sensitive.
A large number of biochemical reactions give rise to a change in charged species in solution, so the majority of reactions can be measured by using conductivitry.
So what are the disadvantages?
Firstly, the measurement does not tell one what the charges are; they are ‘just’ charges giving no information about the chemical structure. However in biochemical experiments (e.g. in assessing the effectiveness of the action of a drug, which is an enzyme inhibitor) one knows the structures of the reactants and products & can therefore interpret what is happening in terms of changes in charges as the reaction takes place.
Spectroscopy, in contrast, does give information about the structure of the molecules involved & has been the favoured method for measuring reactions since early in the 20th century. It is also sensitive and not temperature dependent (although the rate of reaction is). The disadvantage of spectroscopy in many circumstances is that the reaction is often ‘transparent’ in it’s natural form & so produces no change in optical absorbance.
Much ingenuity has been applied in altering the bio-chemicals involved in the reaction in order to make them ‘visible’ in the spectrometer but this frequently also means that the reaction is no longer the fully ‘natural’ one.
TEMPERATURE
By far the most important consideration, and the reason conductivity has hardly been used to measure biochemical reactions, is the high sensitivity to temperature change seen in conductivity measurements. This has previously required the use of very expensive temperature control systems. Because of this it proved necessary to spend a lot of time addressing this issue, in order to make a low cost apparatus which works well.
The result, after many iterations, is an air-circulating system that uses proportional control rather than the usual ‘ON/OFF’ type design. A proportional system provides heat that is proportional to the difference between the target value and the actual value & does not oscillate much. It is accurate to +/- 0.02 deg. C, which has proved sufficient for this purpose & cost about £20. The downside is that it uses more energy than an ON/OFF system.
In biochemistry labs it is usual to use systems that both heat & cool, these cost about £1000 & are accurate to +/- 0.01 deg. C. The disadvantage of the present system is that the ambient temperature has to be significantly below the target temperature. This could be a serious problem away from temperate climes as the measurement temperature is often 25 deg. C. This however can usually be increased to around 40 deg. C without problems. A simple cooling system could be added to maintain 25 deg as will be discussed later in 'For the Future'
The reason for using two conductivity cells (see ac bridge scheme in Instrument Design below) is that it is normally more sensitive and accurate to measure a difference than an absolute quantity, particularly if the difference is small compared with the absolute values.
Thus one of the cells is used as a ‘Reference’ and the other as a ‘Sample’ cell. The contents are as closely matched as possible (i.e. the reference cell lacks only the very low concentration of enzyme) since a bridge of this type is most sensitive near the ‘matched’ or balance position.
A number of reactions have been measured but the one which has given the best results is the urease system & some results are shown in the Results Section.
The urease reaction is:
Urea Urease ammonium ion
NH2 – CO – NH2 + H2O -----> 2NH3 + CO2 -----> 2 x NH4+ + HCO3-
bicarbonate ion
where the ammonia & carbon dioxide produced initially, react in water at pH7 to give the charged ammonium and bicarbonate ions. Thus three charges (2 ammonium ions (+charge) & one bicarbonate (-charge)) are produced for each urea molecule hydrolysed, making it a sensitive assay. The urease assay is important in the analysis of urine, prior to addition of the enzyme the conductivity also informs about the salt content of the urine.
Other assays on the urine can also easily be implemented to allow most of the measurements that can be made using a commercial urine analyser (cost £10k -£100k, depending on how many parameters are determined & the turnover of assays). The instrument presented here would, of course, be much slower as commercial machines in hospital labs are fully automated.
Below is presented a description of the apparatus and two applications. It may have applications in developing countries in medical testing or in school/university teaching labs. The total cost (excluding the computer) is about £200 less if a simpler A/D converter is used. TOP